Cosmic rays bombard the Earth every second, unleashing a cascade of particles that challenge our understanding of physics. Among these particles, muons stand out, presenting a paradox that has fascinated scientists for decades. In this exploration, we will dive into the heart of this mystery and shed light on how it provides compelling evidence for the theory of special relativity.
What Are Cosmic Rays?
Cosmic rays are energetic particles from outer space, primarily protons, that strike the Earth’s atmosphere. They are omnipresent and potent, yet their origins remain somewhat elusive. When these cosmic particles collide with atoms in our atmosphere, they produce a plethora of secondary particles, creating what is known as an atmospheric shower.
The Puzzling Existence of Muons at Sea Level
Muons, which are produced by cosmic ray collisions, are heavier cousins of electrons and have a perplexingly short lifespan—merely 2.2 microseconds on average. Given this ephemeral existence and considering the speed of light, muons should theoretically decay long before they reach the Earth’s surface. Yet, our detectors are brimming with these particles. How can this be?
The Muon Lifespan and Decay
To comprehend the muon paradox, it’s crucial to understand the concept of a half-life. With a half-life of approximately 1.5 microseconds, a population of muons would halve after this duration. However, we detect far more muons on the ground than this rate of decay would allow.
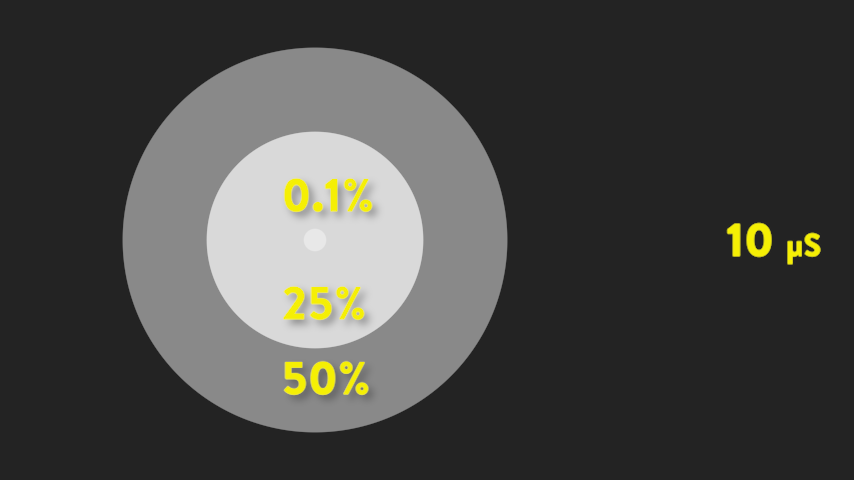
The Journey of Muons: A Closer Look
Understanding the muon paradox requires delving into the physics of these particles’ journey from their creation to their detection:
- Production of Muons: Muons are born high in the atmosphere, around 15 kilometers above the Earth’s surface, when cosmic rays collide with atmospheric particles. This altitude provides them with a considerable distance to cover before reaching the surface.
- Decay Process: As unstable particles, muons spontaneously decay into electrons, positrons, and neutrinos. This decay is random and can happen at any time after a muon is formed, but the probability of decay increases with time, described by the particle’s half-life.
- Detection Techniques: Particle detectors use various methods to identify muons, such as scintillation counters or Cherenkov radiation detectors. These instruments allow physicists to measure the muon flux at the Earth’s surface, providing data to quantify the paradox.
The Resolution Through Time Dilation
The answer lies in time dilation, a cornerstone of Albert Einstein’s special theory of relativity. As muons travel at velocities approaching the speed of light, time for them dilates; it stretches. For a muon zipping through the atmosphere at 99.5% the speed of light, 2.2 microseconds from its perspective equates to about 22 microseconds from ours. This extension allows these fleeting particles to journey significantly farther than we would anticipate without relativistic effects.
Experimental Evidence for Time Dilation
The continuous detection of muons from cosmic rays at ground level serves as robust experimental evidence for time dilation. High-energy muons, traveling even closer to the speed of light, experience greater time dilation, allowing them to cover distances up to or beyond 66 kilometers before decaying.
The Significance of Special Relativity in the Muon Paradox
Special relativity is not just a theoretical concept; it has practical implications and real-world evidence:
- Global Positioning System (GPS): The principles of relativity are employed in the GPS technology we use every day. Without accounting for time dilation effects, the accuracy of GPS would be significantly compromised.
- Particle Accelerators: Experiments in particle accelerators, such as those conducted at CERN, regularly observe time dilation effects in particles traveling at high speeds, similar to cosmic muons.
Length Contraction: The Muon’s Perspective
From the muon’s frame of reference, the phenomenon is explained by length contraction. As the Earth hurtles towards these particles at relativistic speeds, distances contract. To a muon, the Earth’s atmosphere appears much thinner, merely 500 meters, permitting it to traverse the reduced space before it decays.
The Role of Particle Detectors in Understanding Cosmic Rays
Ground-based particle detectors are instrumental in studying cosmic rays and their byproducts. By analyzing the trajectories and energies of secondary particles, scientists can infer properties of the primary cosmic rays. These observations have been crucial in confirming the validity of relativity through the behavior of muons.
Implications of the Muon Paradox
The muon paradox does more than just confirm relativity. It challenges us to rethink our perceptions of time and space. The implications extend to various fields, including astrophysics, particle physics, and cosmology, influencing our understanding of the universe’s structure and the fundamental laws governing it.
Broader Implications of the Muon Paradox
The muon paradox touches on several aspects of modern physics and cosmology:
- Cosmic Ray Sources: Understanding muons helps in the study of cosmic rays, which are still mysterious in their origins. Some theories suggest they emanate from supernovae or black holes, while others propose more exotic sources like dark matter annihilations.
- Astrophysical Observations: The study of muons contributes to astrophysics, especially in the understanding of high-energy processes in the universe. Muons can be used to probe the internal structure of celestial objects, such as the Sun or distant galaxies, through muon tomography.
- Fundamental Symmetries in Physics: The behavior of muons, including their interactions and decay patterns, tests fundamental symmetries in physics, such as charge-parity (CP) symmetry, which is crucial to understanding the matter-antimatter imbalance in the universe.
Advanced Concepts Related to the Muon Paradox
To appreciate the full scope of the muon paradox, it is important to explore advanced concepts that go beyond the basics of relativity:
- Quantum Field Theory (QFT): QFT is the theoretical framework that combines quantum mechanics with special relativity. It provides a deeper understanding of particle interactions, including the creation and decay of muons.
- The Twin Paradox: The muon paradox is often compared to the twin paradox, a thought experiment in special relativity. In this scenario, a twin traveling at relativistic speeds ages more slowly than their sibling on Earth, similar to how muons ‘live longer’ as they hurtle through the atmosphere.
- Muon g-2 Experiment: The muon g-2 experiment measures the anomalous magnetic dipole moment of the muon, which can reveal new physics beyond the standard model, potentially providing insights into the very foundations of particle physics.
The Future of Muon Research
As technology advances, so does our ability to study muons and their paradoxical behavior:
- Next-Generation Detectors: Upcoming technologies aim to detect muons with even greater precision, allowing for more accurate tests of relativity and searches for new physics phenomena.
- Muon Colliders: Future particle accelerators may use muons instead of protons or electrons, thanks to their heavier mass and reduced energy loss through synchrotron radiation, opening new avenues in high-energy physics research.
- Interdisciplinary Research: The study of muons is becoming increasingly interdisciplinary, intersecting with fields such as materials science, geology, and archaeology through techniques like muon tomography, which can image the internal structures of large objects or geological formations.
FAQs on the Muon Paradox
Q: What is a muon?
A: A muon is an elementary particle similar to an electron but with a much greater mass. It is a type of lepton that is produced in high-energy cosmic ray interactions in the Earth’s atmosphere.
Q: Why is the muon’s lifespan considered paradoxical?
A: The muon’s lifespan is paradoxical because, based on its decay rate, it should not survive long enough to reach the Earth’s surface from the upper atmosphere. However, many muons are detected at sea level, which contradicts this expectation if one does not consider the effects of relativity.
Q: What is time dilation, and how does it relate to muons?
A: Time dilation is a concept from Einstein’s theory of special relativity that describes how time can pass at different rates for observers in different reference frames. For muons traveling close to the speed of light, time dilation allows them to live longer from our perspective, enabling them to reach the Earth’s surface before decaying.
Q: What is length contraction?
A: Length contraction is another phenomenon predicted by special relativity, where objects appear to be shortened along the direction of motion relative to the observer’s frame. From a muon’s perspective, the Earth’s atmosphere is contracted, making the distance it has to travel before decaying seem much shorter.
Q: How do we detect muons on Earth?
A: Muons are detected using specialized instruments such as scintillation detectors, cloud chambers, or Cherenkov detectors. These instruments are sensitive to the high-energy particles produced when muons interact with matter.
Q: Can the muon paradox be used to prove the theory of special relativity?
A: Yes, the muon paradox is considered one of the experimental confirmations of the theory of special relativity, particularly the phenomena of time dilation and length contraction.
Q: How do muons contribute to our understanding of the universe?
A: Muons help us study the properties of cosmic rays and provide evidence for relativistic effects predicted by the theory of special relativity. They also have applications in various fields like geophysics and materials science through techniques such as muon tomography.
Q: Are there any practical applications of the muon paradox?
A: While the muon paradox itself is a fundamental physics concept, the principles it confirms (relativistic effects) have practical applications in technologies like the Global Positioning System (GPS), which must account for time dilation to maintain accuracy.
Q: What future experiments might shed more light on the behavior of muons?
A: Future experiments in particle physics, especially those involving high-energy muon beams or muon colliders, as well as further studies of cosmic rays, may provide more insights into the properties of muons and their interactions with other particles and forces.
Q: How fast are muons traveling when they reach the Earth’s surface?
A: Muons created by cosmic rays can travel at speeds up to 99.995% of the speed of light when they reach the Earth’s surface.
Q: Can muons be created artificially, not just in cosmic ray interactions?
A: Yes, muons can be and are regularly created in particle accelerators during high-energy collisions of protons and other particles. This controlled setting allows scientists to study muons and other fundamental particles in great detail.
Concluding Thoughts on the Muon Paradox
The muon paradox provides an elegant demonstration of relativity in action. It shows us that the universe operates on principles that defy our everyday experiences. As we continue to observe these cosmic messengers, we not only unravel the secrets of cosmic rays but also appreciate the profound insights they offer into the fabric of spacetime.
In sum, the muon paradox is not just a quirk of particle physics—it’s a gateway to a deeper understanding of the cosmos. It’s a testament to human ingenuity and curiosity, allowing us to peer into the fundamental workings of the universe from our terrestrial vantage point. As we continue our scientific journey, the paradox of the muon remains one of nature’s most beautiful and mind-bending puzzles, reminding us that reality is often stranger and more spectacular than fiction.